Quantum Hardware
The new horizons of elementary particle
and astrophysics experimentation
being opened up by qubits

Overview
In recent years, there has been growing competition to develop quantum computers, and tremendous progress has been made on qubits and peripheral devices, which are the keys to this development. For example, the lifetime of a superconductive qubit has been increased to one millisecond, an improvement of six orders of magnitude compared to 20 years ago. This barrier has now been surmounted, and qubit readout mechanisms use ultra-low temperatures made possible using dilution refrigerators and quantum amplifiers that leverage the nonlinearity of Josephson junctions to reduce thermal noise to the milli-Kelvin level. External electromagnetic field noise is almost entirely blocked by multiple shields and RF filters. These state-of-the-art quantum technologies, are primarily being developed for use in quantum computers, but they also are highly applicable to elementary particle and astrophysics experiments. ICEPP serves as a bridge between the quantum field and the fields of elementary particles and space, continuously creating new next-generation experiments.
The Quantum Hardware Group primarily engages in research in three areas. The first is "Quantum Sensor Research." Superconductive qubits are sensitive enough to manipulate quanta, the smallest unit of energy, so there are hopes for their use in new sensors that assist in next-generation elementary particle and space experiments. For example, we are applying them to the search for dark matter. We are taking the lead in experiments such as tuning and single photon detection experiments that use the mutual interaction between qubits and resonant cavities ("containers" for enclosing electromagnetic fields) and experiments in which we use quantum entanglement to perform direct excitation of dark matter. Gravitational waves are normally detected using large laser interferometers, but for high frequencies exceeding the kHz band, we have found that they can be detected more efficiently using resonant cavities and qubits. That is why we are carrying out high frequency gravitational wave detection research that uses accelerator cavities and qubits.
The second is "Quantum Bit Peripheral Device Development." The number of qubits in quantum computers has reached several thousand, but error correction is said to require one million bits. The peripheral devices to be used with quantum computers are still in the midst of development, so it is urgent that compact, high performance, mass-producible components are developed. We are working closely with private sector companies such as IBM to carry out wide-ranging hardware research for producing workable quantum computers.
The third research field is "Research into Extreme Physical Phenomena Using Mathematical Analogy." As Richard Feynman, one of the giants of modern physics, put it, "Nature isn't classical, dammit, and if you want to make a simulation of nature, you'd better make it quantum mechanical." This idea is said to have kicked off quantum computer research. Indeed,
with new emulators that apply quantum computers and their principles, it is possible to artfully use mathematical analogies to verify space phenomena, such as black holes and Hawking radiation, which only occur in extreme situations. We are opening up the new research field of quantum space-time using light and meta-materials, and we are developing new superconductive circuits and qubits with unique properties to replicate Hawking radiation.
This research is relative new, seeing rapid growth in the past few years, so collaboration with experts in various fields is vital. Our center works together with those at the forefront of elementary particle and quantum information theory, quantum technologies, and accelerator research, conducting research with their solid backing. These research trends are occurring not only in Japan but also overseas, so we are actively engaging in joint international research. Collaboration with a wide range of joint researchers, such as U.S.-based Fermilab and the University of Chicago, EPFL in Switzerland, and other organizations, provides us with access to state-of-the-art research facilities.
What are superconductive qubits?
Superconductive qubits are electronic circuits in which the inductors (L) of LC resonant circuits are nonlinear. This nonlinearity is derived from Josephson junctions, which consist of an insulator sandwhiched between two superconductors. In a normal LC resonant circuit, energy levels are equally spaced, but the nonlinearity of Josephson junctions causes the energy levels of qubits to be unevenly spaced. This property can be used in selective operation, from the smallest energy level (|0> state) to the next energy level (|1> state). Josephson junctions are typically made from aluminum and function at temperatures below their superconducting transition temperature (1.2 Kelvin). Their sizes are on the order of 100 nm, and they can be fabricated using a similar process to the semiconductor processes used in CPUs, etc.
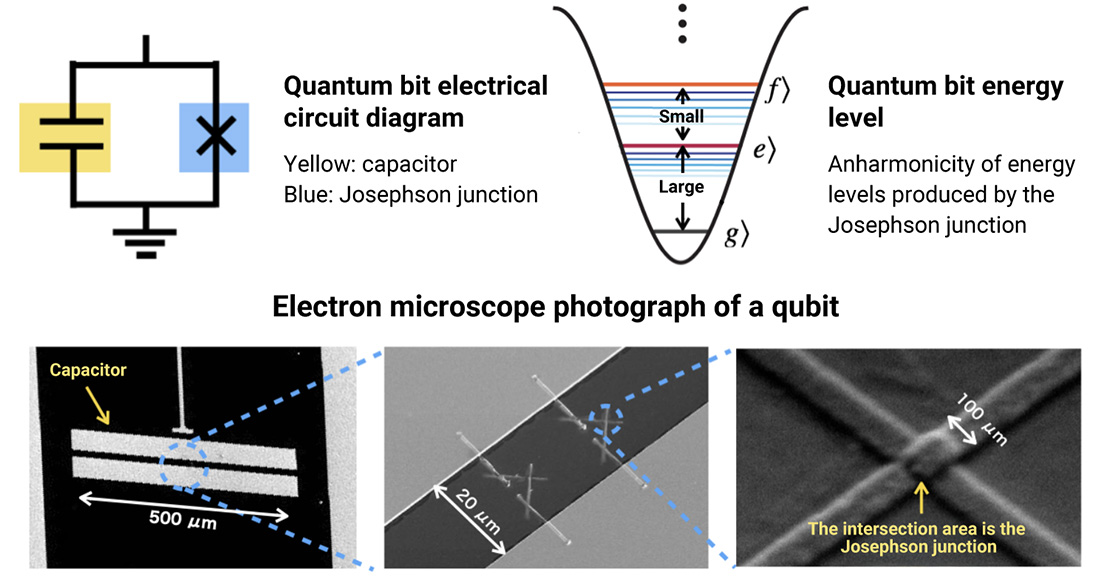